Research
Introduction
The Martin lab is interested in how tissues get into shape. Understanding tissue shape requires understanding how cells generate force and work together to collectively sculpt a tissue. One system that a cell uses to generate force is the actin-myosin cytoskeleton; a network composed of the molecular motor myosin, which slides actin filaments together to pull on boundaries of the cell (contractile force). We have elucidated how cells generate force and how this force is propagated to the tissue-level to fold a tissue. We continue to study how tissue integrity is regulated and also investigate mechanisms that regulate the epithelial-to-mesenchymal transition or EMT and cell division orientation. We do so using fruit fly and mouse model systems. We have an ongoing collaboration with experts in physical mechanisms of pattern formation and mathematical modeling (Jörn Dunkel’s lab, Math) to learn new things and solve interdisciplinary problems.
Research in the Martin Lab falls in the following four categories: 1) Tissue Folding, 2) Cell Mechanics and Signaling in Form, 3) Epithelial-mesenchymal Transition, and 4)Cell Division Orientation and Morphogenesis.
1. Tissue Folding
a) Folding as a Mechanism for Morphogenesis – Fruit Fly Gastrulation and Mouse Neural Tube
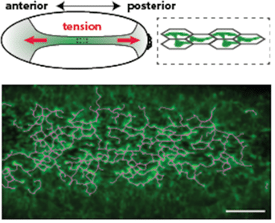
Much like folding paper generates more complex forms, two-dimensional sheets of polarized cells called epithelia can be folded to sculpt tissues. Actomyosin contractility is associated with tissue folding, tube formation (e.g., the neural tube), and cell extrusion from epithelial layers. Our past work uncovered cellular mechanisms responsible for generating contractile force. Our current work aims to understand how cells work together to achieve dramatic shape changes, such as tissue folds.
b) Transmitting Forces Across a Tissue
For contractility to change tissue shape, forces have to be transmitted between cells in the tissue. Adherens junctions, which contain the transmembrane adhesion molecule E-cadherin, link neighboring epithelial cells to each other. In addition, force transmission requires that the actin cortex is linked to the adherens junctions. Current projects in the lab include understanding how actin becomes attached to adherens junctions and how adherens junctions themselves are patterned across the embryo.
Using live imaging, we have shown that actomyosin forms a supracellular network that is coupled between cells by adherens junctions (Figure 1). We discovered that: 1) genes involved in actin turnover are critical to maintain intercellular connections during folding, with disruption of turnover leading to dramatic network failure (see Movie); we continue to examine the regulation of actin assembly and disassembly, 2) redundancy in the organization of the supracellular actomyosin network confers robustness to local damage in the network, and 3) Forces that are transmitted across the tissue can feed-back on cells to regulate their behavior and actually orient the cytoskeleton and force generation.
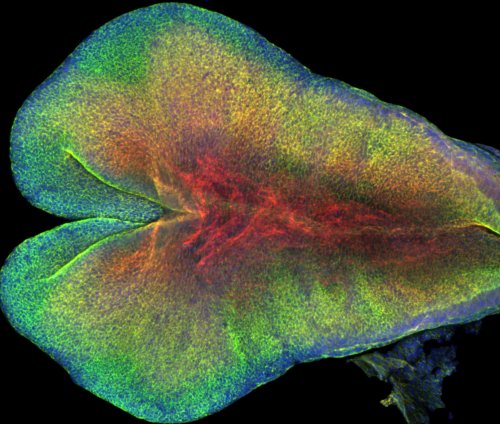
Our results provided insight into the process of mammalian central nervous system formation, specifically folding of the neural tube. Neural tube defects result in a number of devastating birth defects, such as anencephaly and spina bifida. Current work in the lab aims to map the patterns of mechanical interactions across the tissue that lead to neural tube closure in mice (Figure 2). In addition, we are studying the mechanical basis of neural tube defects.
c) The Interplay Between Cell Signaling and Form
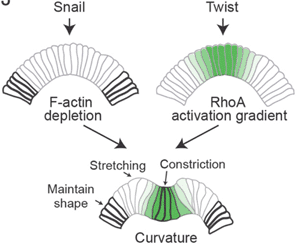
The RhoA GTPase is a key signaling molecule that regulates actomyosin. Active RhoA leads to both myosin activation and actin filament assembly. RhoA is activated by Guanine nucleotide exchange factors (GEFs) and inactivated by GTPase activating proteins (GAPs). Precise regulation of RhoA is required at both the cell and tissue level for fold a tissue.
Using a reverse genetic screen, we identified a RhoGAP that is expressed in embryonic furrow, which we named Cumberland GAP (C-GAP). C-GAP is required for the proper spatial and temporal control of RhoA and myosin. This uncovered a surprising role for a RhoA inhibitor promoting contractility.
Contractility must also be temporally and spatially regulated across the tissue. Myosin accumulates as a gradient across the tissue whereas actin filaments initially become depleted across the primordium (Figure 3). This contractile gradient is important for folding and is the result of a gradient in gene expression. We found that the negative regulation of RhoA by C-GAP is critical to refine this pattern and regionalize the tissue into zones that contract and stretch.
2. Cell Mechanics and Signaling in Form – Egg Cell Formation
Egg cells need to be stocked with the cellular materials needed to get to a stage of development where zygotic gene expression begins. One strategy that organisms use to provide material for the egg cell is for support cells to transport cytoplasm into the developing egg cell (oocyte). In fruit flies, oocytes develop as part of a multicellular network where cells are interconnected through cytoplasmic bridges and attached support cells are called nurse cells. We discovered that nurse cells ‘dump’ their cytoplasm into eggs in a stereotypic fashion, where uniform actomyosin contractility initiates cytoplasmic flow into oocyte and then transition to dynamic actomyosin waves that squeeze remaining cytoplasm into oocyte like emptying a tube of toothpaste.
Like tissue folding, the lab identified a RhoGAP that is required for the precise regulation of actomyosin dynamics in this process. We are currently using both the fruit fly early embryo and the nurse cell/oocyte systems to understand how RhoA is dynamically regulated and coupled to cell shape/mechanics.
3. Epithelial-mesenchymal Transition
Apical constriction can constrict cells and fold tissues, but it can also cause cells to extrude from an epithelium. What determines which of these two outcomes occurs? In a genetic screen, we discovered that depleting the Abelson non receptor tyrosine kinase causes cells that normally undergo apical constriction to abnormally extrude from the epithelium during tissue folding. Interestingly, this extrusion is associated with an EMT-like loss of apical-basal polarity. The EMT-like loss of apical-basal polarity and extrusion in Abelson mutants depends on the gene Enabled. Enabled is implicated in cancer cell metastasis. Our work has identified similar cell extrusion phenotypes for other genes that play a role in force generation and we are searching for the link between these disparate signaling pathways.
4. Cell Division Orientation and Morphogenesis
Cell division is critical to grow a tissue. Epithelial sheet growth requires that cell division occurs in the plane of the sheet. We are studying the forces that allow cell division to occur in the correct orientation to grow an epithelium. To address this problem, we are utilizing live imaging of the early Drosophila embryo to directly visualize cell divisions and observe cell shape changes during mitosis.
During embryonic development, cell division must be coordinated with tissue shape changes to ensure proper form. The Martin lab is interested in the interplay between various processes that happen during cell division and those that drive tissue shape change. In some cases, it appears that cell division can interfere with morphogenetic processes that change cell shape and we are investigating how these processes are coordinated and what happens when they are not.
Resources
Below are Drosophila and molecular cell biology resources.